the Creative Commons Attribution 4.0 License.
the Creative Commons Attribution 4.0 License.
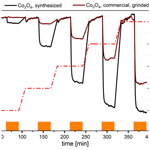
Impact of particle size and morphology of cobalt oxide on the thermal response to methane examined by thermal analysis
Olena Yurchenko
Hans-Fridtjof Pernau
Laura Engel
Benedikt Bierer
Martin Jägle
Jürgen Wöllenstein
Differential thermal analysis (DTA) was used to examine the effect of the particle size and morphology of cobalt (III/IV) oxide (Co3O4) on its thermal response under exposure to methane (1 vol % in dry synthetic air), which is a relevant gas for the detection of combustible gases. The DTA response results from the catalytic oxidation of methane, and its characteristics should correlate with the pellistor response. Co3O4 samples differing in particle size and morphology were produced by ball milling (top-down technique) or were synthesized from precursor molecules by precipitation (bottom-up technique). The investigations carried out in dry air and a temperature range between 250 and 450 ∘C reveal that both particle size and particle shape have a considerable effect on thermal response, since the resulting layer structures and the associated surface area available for gas interaction differ. The Co3O4 catalyst, with small particles and an irregular shape, exhibits significantly higher response than milled Co3O4 samples. Comparison of DTA with the mass spectroscopy signal of CO2 evolved by the reaction verified a certain analogy between DTA measurements and the response produced by a pellistor.
- Article
(1666 KB) - Full-text XML
- BibTeX
- EndNote
In the field of safety technology, catalytic sensors, so-called pellistors, are commonly used for the detection of flammable gases such as hydrocarbons. A pellistor typically consists of two sensor elements. One sensor element, called the “detector”, contains the active catalyst and is designed to respond to the target gas. The other sensor element is the “compensator”, manufactured without a catalyst, and only responds to changes in environmental conditions, thus providing an inherent temperature compensation (White, 2014). In the presence of a combustible gas, heat is generated due to the catalytic oxidation of gas on the catalytic layer of the detector. This generates an electrical signal, which corresponds to gas concentration in the environment.
Currently, commercially available pellistors typically operate at high temperatures (>450 ∘C) to ensure the accurate detection of methane, which is the most inert combustible gas (Trautweiler et al., 2012). However, high operating temperatures entail some disadvantages, such as high power consumption and reduced catalyst stability. Reducing the operating temperature should contribute to a decrease in the power consumption and an increase in the sensor's operating time due to a slower aging of the catalyst. Especially for the detection of methane, catalysts of high activity and stability are required for the reduction in the operating temperature.
The choice of suitable catalysts for the targeted gas sensor application is not easy when considered from different points of view. First, the catalytic material used for sensors is a complex system consisting of catalysts and various additives that enable the integration into the sensor and generate the mechanical stability of the catalytic layer. Additives can affect the catalyst activity and stability, and their effect is difficult to identify during the characterization of the gas sensor. Second, the response detected by a sensor is a complex reaction as well, as it is determined by the whole sensor system. Third, the preparation process of individual sensors is quite extensive. This additionally limits a specified evaluation of the effects of physical properties such as the surface area or particle size distribution.
To overcome these limitations existing in investigations of pellistor gas sensors, thermal analysis methods, including differential scanning calorimetry (DSC) or differential thermal analysis (DTA), can be used to perform the preselection of the catalysts (Le Parlouër, 2013; Janssen, 1989; Borchardt and Daniels, 1957). DSC is a well-known technique in catalyst research and widely used for investigation of catalytic reactions (Hakvoort and Xiaoding, 1991; Loskyll et al., 2012; Hakvoort, 1996). In DSC, the heat flux difference to/from the sample, compared to the reference, is recorded versus time, while both are subjected to a controlled temperature program. However, DTA should be more appropriate for the investigation of catalysts for gas sensor purpose, since the temperature difference ΔT between the sample and reference is measured when both are subjected to equal heat fluxes in a controlled atmosphere (Beecroft et al., 1975). The DTA method, therefore, uses a functional principle that is comparable to that used for pellistor measurements. The combination of thermal analysis methods with a mass spectrometry (MS) technique for the analysis of the evolving gases can provide additional complementary information that is useful for investigations (Hakvoort, 1996).
In the present work, we report on the use of a differential thermal analysis system that, in combination with a mass spectrometer, is used for a catalyst examination to define the influence of particle size and morphology on the thermal response. Some kinds of metal oxides, like CuO, Co3O4, MnOx and CeO2 (Chen et al., 2015), can act as catalyst for the oxidation of flammable gases. Thus, spinel Co3O4, which is reported to be a promising catalyst for methane combustion (Pu et al., 2017), was used as noble metal-free catalytic material for the investigations. The size of the catalytic particles has a significant, but sometimes opposite, effect on the catalytic oxidation of methane. Investigations on noble metal catalysts Pt/Al2O3 (Stakheev et al., 2013) and Au/Al2O3 (Grisel et al., 2000) have shown that the reduction in Au particle size, from 44 to 3–5 nm, leads to a rise in the catalytic activity, whereas no significant effect could be observed for Pt/Al2O3 catalyst for Pt particles in the range between 2 and 14 nm. In turn, the intrinsic catalytic activity of a Pd/Al2O3 catalyst considerably increases when the Pd particle size grows from 1 to 20 nm (Stakheev et al., 2013). Also, for Co3O4 catalysts, the influence of morphology and crystallite size on catalytic behavior in methane combustion was reported (Pu et al., 2017; Chen et al., 2016).
The effect of particle size on methane oxidation was systematically investigated at different operating temperatures using Co3O4 catalyst materials that were produced with different milling times (top-down technique) to achieve different particle sizes. To include the effect of morphology in the investigations, intrinsically nano-sized Co3O4 material (bottom-up technique) was synthesized. The thermal response of the methane oxidation was observed using DTA and compared to the mass spectrometer response, which directly reflects the methane conversion.
The commercially available STA–QMS (simultaneous thermal analyzer and quadrupole mass spectrometer; NETZSCH-Gerätebau GmbH; STA 409 CD-QMS 403/5 SKIMMER), equipped with a DTA sample carrier, was adapted for the investigation of catalytic activity at dry conditions. The integrated quadrupole mass spectrometer was used to analyze CO2 species in exhaust gas that evolved during the catalytic reaction. Therefore, the characteristic mass number of CO2 () was measured. In DTA, the temperature difference between the reference and sample crucible, as a result of a thermal event on a sample, is detected as an electrical signal (µV). This signal is normalized by software to the sample weight (µV mg−1), allowing a direct comparison between different samples. Nonetheless, all measurements were performed on the same amount of the catalyst (20 mg) to minimize the influence of additional disturbance variables. Aluminum oxide was chosen as the crucible material because it is inert under the given conditions. An empty crucible of the same size and type was used as reference. Since heat is released during the catalytic oxidation, the DTA signal shows a negative output. For the investigation, the air and methane mixture (1 vol % CH4 in synthetic air; Air Liquide Deutschland GmbH) were automatically switched accordingly to the stated program. The experiments were performed at a constant gas flow rate of 100 mL min−1, regulated by mass flow controllers and isothermal conditions within the temperature range of ∘C. At each temperature level, the baseline recorded in air was set to zero.
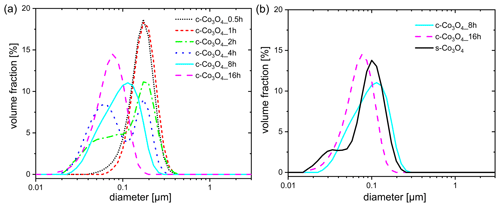
Figure 1Differential volume distribution of particle sizes obtained for (a) commercial Co3O4 samples ground for different durations and (b) two Co3O4 samples ground for 8 and 16 h in comparison to the synthesized Co3O4 sample.
In order to investigate the effect of catalyst particle size, commercially available Co3O4 powder (400 mesh; 37 µm; Alfa Aesar; 99.7 %) was wet ground in a zircon jar by means of a planetary ball mill (PM 100; Retsch GmbH) for different durations (between 0.5 and 16 h). The samples were accordingly denoted as c-Co3O4, with an indication of the milling duration, e.g., a sample milled for 0.5 h was named “c-Co3O4_0.5h”. The final samples were first dried at 130 ∘C for 3 h and then thermally treated at 400 ∘C for 12 h. Additionally, the Co3O4 sample was synthesized by the following precipitating procedure: Co(NO3)2 6H2O (17.46 g; Carl Roth; >98 %) was dissolved in 100 mL deionized water at 23 ∘C. Then, a KOH solution (1 mol L−1, 300 mL) was added under the bubbling of nitrogen gas and continuous stirring. A precipitate was collected and washed three times with hot deionized water (60 ∘C), followed by drying at 130 ∘C for 24 h. The obtained solid was ground to powder in a mortar and further calcined at 350 ∘C in air for 4 h, forming the synthesized catalyst, which was denoted as s-Co3O4. A detailed description of this procedure can be found elsewhere (Tao et al., 2015).
The particle size distribution was determined with a laser particle size analyzer (LB-550; Horiba Instruments). To determine the particle size distribution, a drop of milled c-Co3O4 samples was dissolved in 10 mL ethylene glycol. At least five measurements were performed with each sample solution, since the uncertainty of an individual measurement is relatively high, and the mean value was provided for the presentation as result. A scanning electron microscopy (SEM) investigation was performed on a film deposited from sample dispersions after its thermal treatment at 450 ∘C, using the SU-70 digital elevation model (Hitachi High-Tech Corporation).
For both types of catalysts, a thermal stability test was carried out by alternating between dry synthetic air (30 min) and the methane mixture atmosphere (volume percentage of CH4 in synthetic air; 30 min), firstly at 350 ∘C and then at 450 ∘C, for a total time of 7 h in each case.
3.1 Particle size and morphology of samples
The Co3O4 samples were firstly examined with respect to their particle size distribution by means of a particle size analyzer. The particle size is analyzed with regard to a volume distribution representing the population of particles seen by their volume. The volume-average size is more sensitive to the presence of larger particles. It should be noted that this analysis technique does not provide any information about particle shapes and is more accurate for determining the representative diameter of relatively spherical particles (Burgess et al., 2004). To manufacture the samples with a controlled reduction in particle size, the commercial micro-sized Co3O4 powder was milled for defined durations. For comparison, a Co3O4 sample with initially nano-sized particles was synthesized by the precipitation method.
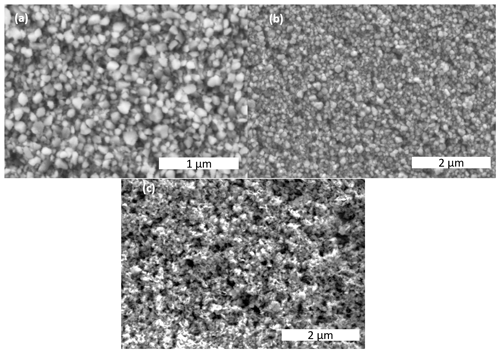
Figure 2SEM images of (a, b) commercial Co3O4 nano powder, after milling for 8 h (c-Co3O4_8h) in different magnifications, and (c) synthesized Co3O4 (s-Co3O4).
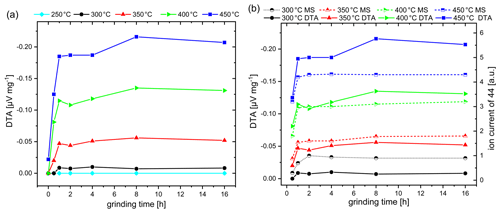
Figure 3(a) DTA response obtained on CH4 exposure (1 vol % in dry air) of commercial Co3O4 catalysts as a function of grinding time and operating temperature. (b) DTA response in comparison to MS response obtained by simultaneous measurements.
The investigation of the particle size distributions of ground samples (Fig. 1a) exhibits that, after milling for 30 min and 1 h (c-Co3O4_0.5h and c-Co3O4_1h), the majority of the total particle volume originates from particles with sizes between 100 and 400 nm, exhibiting a peak at ca. 200 nm. With a continuous increase in the milling time, a second peak appears at lower particle sizes (c-Co3O4_2h; c-Co3O4_4h) and rises over time until the first peak disappears (c-Co3O4_8h; c-Co3O4_16h), evidencing the increase in the volume percentage of small particles. In comparison to Co3O4 samples ground for 8 and 16 h, represented by curves with single maximum at 100 and 80 nm, the synthesized one (s-Co3O4) exhibits a broad curve with two maxima located at 100 and 30 nm, respectively (Fig. 1b). The maximum at 30 nm evidences the presence of smaller particles for the synthesized Co3O4 sample in a high amount.
SEM images of Co3O4 milled for 8 h (Fig. 2a and b) affirm that particles of different sizes are present in the sample. In addition, as an imaging technique, SEM reveals the presence of spherical particles with sharp edges, which is expected for the mechanical breaking of big particles. The particles also form a compact layer. These SEM investigations on the synthesized sample (Fig. 2c) indicated that the particles are not spherical and possess a rather random shape. Additionally, most particles reveal a rather flat shape, which is a significant difference to the milled Co3O4 samples.
3.2 Effect of grain size and morphology on thermal response
Figure 3a illustrates the dependence of the DTA response to commercial Co3O4 on the grinding time in a temperature range between 250 and 450 ∘C, since high temperatures of up to 450 ∘C are usually used for the pellistor operation. The original Co3O4 powder with micro-sized particles exhibits no DTA response to methane up to 400 ∘C. First, at 450 ∘C, a minor signal of −0.022 µV mg−1 is observed. Indeed, increasing the grinding time leads to a successive increase in the DTA response at temperatures starting from 300 ∘C, which is especially noticeable in the first 2 h of grinding. A further increase in the grinding time has no significant effect on improving the catalytic activity. The initial response increase correlates well with reduction in the particle size, as indicated by particle size investigations (Fig. 1a), which should be a result of the increased surface area accessible for gas contact and reaction. The minor increase in the thermal response by the further decrease in particle size can be explained by the particle packing density in the sample powder. With progressive grinding time and an increase in the polydispersity of the system, the packing density of the spherical particles should increase, since small particles can efficiently occupy the voids created by neighboring large particles (Desmond and Weeks, 2014; Elmsahli and Sinka, 2020). The reduction in the particle size increases the cohesion between the particles, which, furthermore, affects the density of the samples (Elmsahli and Sinka, 2020). The porosity decrease, as a result of attractive forces between nano-sized particles, is a possible explanation for the minor effect of continued milling on the DTA response, which counteracts the positive effect of an increased particle surface.
When the DTA signal (solid line) is compared with the MS signal (dashed line) (Fig. 3b; the results for non-milled Co3O4 sample and for 250 ∘C are not included), it is obvious that the grinding time dependence of the MS signal follows the dependence on the DTA signal but with an offset. A significant MS signal can already be observed at 300 ∘C, while the DTA signal is still minor. Moreover, the difference in the signal height of the MS and DTA signal becomes smaller with increasing operating temperature and is reversed at 400 ∘C. In relative terms, the DTA signal is higher than the MS signal at 400 and 450 ∘C. Figure 4a shows the different developments of the DTA (lower half of graph) and the MS curve (upper half of graph) with the example of a Co3O4 sample ground for 8 h. The MS signal already appears at 250 ∘C, while no thermal signal is observed. This deviation can be explained by the fact that some amount of heat evolved by the reaction is required to heat the crucible before the temperature increase can be detected by thermocouples. The deviations in the responses result from the specifics of both methods.
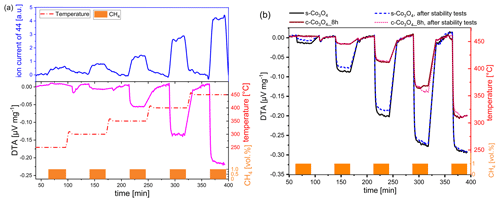
Figure 4(a) DTA curve in comparison to MS curve of ground Co3O4 (8 h) under exposure to CH4. (b) DTA curves for two different Co3O4 catalysts before and after thermal stability investigations. Conditions – pulses of 1 vol % CH4 in dry air for 30 min in a temperature range between 250 and 450 ∘C.
The comparison of the DTA curves of commercial Co3O4 ground for 8 h with one of the prepared Co3O4 (Fig. 4b) reveals that synthesized Co3O4, with initially nano-sized particles and a random shape, shows considerably higher activity (black line). At 350 ∘C, the DTA response of synthesized Co3O4 is three times higher compared to ground Co3O4, with −0.18 µV mg−1 vs. −0.06 µV mg−1, respectively, while, at 450 ∘C, the DTA signal ratio is only 1.3 for both catalysts. This points out that, at high operating temperatures, the advantage of a catalyst with high catalytic activity is less pronounced than at low operating temperatures.
The considerably higher activity of synthesized Co3O4 can be explained, on the one hand, by its lower particle size (Fig. 1b) and, on the other hand, by differences in the morphology of both samples, since the particle shape is a further key factor affecting the packing of the material and its porosity. Irregularities in the particle shape influence the interparticle friction, lowering the packing density (Elmsahli and Sinka, 2020). The irregular particles can be assembled in a less dense configuration, with higher porosity and bigger surface area accessible for gas interactions. Future investigations should clarify to what extent the particle shape has an influence on DTA response.
The results of the thermal stability test are shown for both types of catalysts in Fig. 4b. Synthesized Co3O4 exhibits a slightly lower thermal stability than the commercial one. The decrease in activity after thermal treatment in a methane-containing atmosphere is particularly observed at lower operating temperatures (≤350 ∘C), which are more interesting for pellistor applications. The reason for the decreased activity of synthesized Co3O4 originates most probably from its loosely packed morphology and the sintering of nanoparticles occurring at high operating temperatures (450 ∘C). In addition, small particles are more susceptible to sintering than large particles (Hakvoort and Xiaoding, 1991).
Differential thermal analysis (DTA) was used to examine the effect of the morphology of Co3O4 catalysts on the thermal response towards methane gas with regard to pellistor applications. The evaluation was performed by means of ground and synthesized Co3O4 catalysts. It has been demonstrated that, in addition to particle size, the particle shape also plays an essential role in determining the thermal response by influencing the packing of the particles in the layer and, therefore, the surface area of the catalyst. The initially nano-sized Co3O4 sample with an irregular shape of nanoparticles had a considerably higher thermal response than ground samples with approximately spherical particles and an increased tendency to form compact layers. Consequently, the investigations demonstrate the limits of the milling method for increasing the surface area and improving the sensor sensitivity. Furthermore, the morphology of Co3O4 catalysts had a higher impact on the improvement of the response than raising the temperature. Nevertheless, the effect of the catalyst activity was less pronounced at 450 ∘C than at 350 ∘C, which is the most interesting temperature for pellistor applications. The thermal stability of nano-sized Co3O4 particles, as determined after successive measurements at 450 ∘C, is slightly lower due to the sintering process occurring. The influence of the morphological parameters should be investigated in more detail in further work. Finally, the thermal response from the DTA was compared with the signal of evolved carbon dioxide as an oxidation product by means of mass spectrometry (MS), which was integrated into a measurement system. The deviation of the signals detected by DTA and MS was explained by the principle of the thermal method.
All relevant data presented in the article are stored in a public data repository, Fordatis, under https://doi.org/10.24406/fordatis/118 (Yurchenko et al., 2021).
JW created the concept for pellistor development and was responsible for the funding acquisition. LE prepared the Co3O4 samples from commercial metal oxide and conducted the particle size examinations. OY carried out the synthesis of Co3O4 sample, performed the SEM and DTA investigations and wrote the original draft. The concept of DTA method as a tool for catalyst screening was developed by OY and HFP in close discussion with JW, MJ and BB. HFP was responsible for the DTA measurement instrumentation. All authors contributed to the reviewing and editing of the final paper.
The authors declare that they have no conflict of interest.
This article is part of the special issue “Sensors and Measurement Science International SMSI 2020”. It is a result of the Sensor and Measurement Science International, Nuremberg, Germany, 22–25 June 2020.
This work was supported by the Fraunhofer Society as part of the FluMEMs research project and also the Sustainability Center Freiburg as part of the LeakAlert project.
This research has been supported by the Fraunhofer Society (grant FluMEMS).
This paper was edited by Jens Zosel and reviewed by two anonymous referees.
Beecroft, T., Miller, A. W., and Ross, J. R. H.: The use of differential scanning calorimetry in catalyst studies. The methanation of carbon monoxide over nickel/alumina catalysts, J. Catal., 40, 281–285, https://doi.org/10.1016/0021-9517(75)90255-9, 1975.
Borchardt, H. J. and Daniels, F.: The Application of Differential Thermal Analysis to the Study of Reaction Kinetics, J. Am. Chem. Soc., 79, 41–46, https://doi.org/10.1021/ja01558a009, 1957.
Burgess, D. J., Duffy, E., Etzler, F., and Hickey, A. J.: Particle size analysis: AAPS workshop report, cosponsored by the Food and Drug Administration and the United States Pharmacopeia, AAPS J., 6, 20, https://doi.org/10.1208/aapsj060320, 2004.
Chen, J., Arandiyan, H., Gao, X., and Li, J.: Recent Advances in Catalysts for Methane Combustion, Catal. Surv. Asia, 19, 140–171, https://doi.org/10.1007/s10563-015-9191-5, 2015.
Chen, Z., Wang, S., Liu, W., Gao, X., Gao, D., Wang, M., and Wang, S.: Morphology-dependent performance of Co3O4 via facile and controllable synthesis for methane combustion, Appl. Catal. A, 525, 94–102, https://doi.org/10.1016/j.apcata.2016.07.009, 2016.
Desmond, K. W. and Weeks, E. R.: Influence of particle size distribution on random close packing of spheres, Phys. Rev. E, 90, 22204, https://doi.org/10.1103/PhysRevE.90.022204, 2014.
Elmsahli, H. S. and Sinka, I. C.: A discrete element study of the effect of particle shape on packing density of fine and cohesive powders, Comp. Part. Mech., 20, 155, https://doi.org/10.1007/s40571-020-00322-9, 2020.
Grisel, R. J. H., Kooyman, P. J., and Nieuwenhuys, B. E.: Influence of the Preparation of Au/Al2O3 on CH4 Oxidation Activity, J. Catal., 191, 430–437, https://doi.org/10.1006/jcat.1999.2787, 2000.
Hakvoort, G.: Measurement of heterogeneously catalyzed gas reactions by DSC, J. Therm. Anal., 47, 445–452, https://doi.org/10.1007/BF01983986, 1996.
Hakvoort, G. and Xiaoding, X.: Decomposition of methanol vapour over solid catalyst, measured by DSC, J. Therm. Anal., 37, 1269–1279, https://doi.org/10.1007/BF01913860, 1991.
Janssen, F.: The use of thermal analysis techniques in heterogeneous catalysis, Thermochim. Acta, 148, 137–147, https://doi.org/10.1016/0040-6031(89)85210-4, 1989.
Le Parlouër, P.: Thermal Analysis and Calorimetry Techniques for Catalytic Investigations, in: Calorimetry and Thermal Methods in Catalysis, vol. 154, edited by: Auroux, A., 52 pp., Springer, Berlin, Heidelberg, https://doi.org/10.1007/978-3-642-11954-5_2, 2013.
Loskyll, J., Maier, W. F., and Stoewe, K.: Application of a Simultaneous TGA-DSC Thermal Analysis System for High-Throughput Screening of Catalytic Activity, ACS Comb. Sci., 14, 600–604, https://doi.org/10.1021/co3000659, 2012.
Pu, Z., Zhou, H., Zheng, Y., Huang, W., and Li, X.: Enhanced methane combustion over Co3O4 catalysts prepared by a facile precipitation method: Effect of aging time, Appl. Surf. Sci., 410, 14–21, https://doi.org/10.1016/j.apsusc.2017.02.186, 2017.
Stakheev, A. Y., Batkin, A. M., Teleguina, N. S., Bragina, G. O., Zaikovsky, V. I., Prosvirin, I. P., Khudorozhkov, A. K., and Bukhtiyarov, V. I.: Particle Size Effect on CH4 Oxidation Over Noble Metals: Comparison of Pt and Pd Catalysts, Top. Catal., 56, 306–310, https://doi.org/10.1007/s11244-013-9971-y, 2013.
Tao, F. F., Shan, J.-J., Nguyen, L., Wang, Z., Zhang, S., Zhang, L., Wu, Z., Huang, W., Zeng, S., and Hu, P.: Understanding complete oxidation of methane on spinel oxides at a molecular level, Nat. Commun., 6, 65, https://doi.org/10.1038/ncomms8798, 2015.
Trautweiler, S., Moser, N., Delprat, H., Lepoutre, S., Alépée, C., Brown, T., and Brown, K.: Disruptive MEMS Technology Replaces Conventional Bead Pellistor Device, in: IMCS 2012, 20 May 2012, Nuremberg, Germany, https://doi.org/10.5162/IMCS2012/3.4.3, 2012.
White, R.: The pellistor is dead? Long live the pellistor! 2014, available at: https://www.envirotech-online.com/article/environmental-laboratory/7/sgx-sensortech/the-pellistor-is-dead-nbsplong-live-the-pellistor/1699 (last access: 26 January 2021), 2014.
Yurchenko, O., Engel, L., and Pernau, H.-F.: Impact of particle size and morphology of cobalt oxide on thermal response to methane examined by thermal analysis, fordatis, https://doi.org/10.24406/fordatis/118, 2021.